In 2023, wind turbines generated upwards of 425,000 gigawatt-hours of electricity in the United States, enough to run more than 39 million average American homes. Wind is the country’s largest renewable energy source, representing about 10% of all electricity production.
Most of it comes from land-based wind turbines; the remainder, a negligible amount, comes from offshore turbines along the East Coast. Yet our offshore wind energy has barely been tapped, according to the Wind Energy Technologies Office, which estimates that potential U.S. offshore wind power capacity exceeds 4,200 gigawatts, or 13,500 terawatt-hours annually. That’s three times the amount of electricity consumed every year in the United States.
“Wind over land can be disrupted by terrain, buildings, trees, and other irregularities that slow it down and diminish its quality as an energy source,” said Bryson Robertson, professor of coastal and ocean engineering and director of the Pacific Marine Energy Center. “These aren’t factors with offshore wind.”
There is a catch: Two-thirds of those unimpeded offshore winds blow over West Coast waters ranging from 500 to 1,500 meters deep. Fixed-bottom wind turbines are limited to depths of about 60 meters. Anything deeper and they must be mounted on floating platforms moored to the seabed — a more technically challenging and more costly solution.
Floating wind turbines
A handful of floating wind turbines have been operating in European waters for several years, most prominently in the North Sea. Their combined power output is modest (though it’s on the rise), and they operate in water depths ranging from 60 to 300 meters, but which skew toward the shallower end.
“The water is much deeper off the West Coast; the waves are larger, longer, and more energetic,” said Pedro Lomonaco, director of Oregon State’s O.H. Hinsdale Wave Research Lab and a research team member. “Those factors have to be considered when designing and testing floating platforms. The designs used in the North Sea may be suitable for those locations, but they may not be optimal for our area.”
But designing, building, deploying, and testing operating prototypes is prohibitively expensive, logistically difficult, and time-consuming. Scaled-down simulations offer a more flexible and affordable alternative, Robertson explains.
Offshore wind energy research at Oregon State
“We want to be able to test the technology quickly, accurately, and inexpensively without the need to build multimillion-dollar machines and test them in the ocean,” he said. Robertson is the co-principal investigator of a study to explore the effectiveness of coupling physical experiments with numerical modeling. The resulting hybrid simulation was used to evaluate the performance of a floating wind turbine model at the Hinsdale wave lab. “There’s no reason researchers can’t come here to test a variety of different floating platform configurations to understand their performance characteristics.”
In the study, Robertson and his colleagues built a 1/50 scale model of a floating wind platform. The model includes the turbine mast but not the blades. They subjected the tripodal, semi-submersible design to a range of sea conditions in the wave lab’s multidirectional wave tank.
Results of the open-source study, funded by the U.S. Department of Energy, will be made available to anyone.
“We first needed to determine what hydrodynamic tests to run in the wave tank. Then we had to figure out the best ways to measure and characterize the model’s responses so that the results could be used to predict how a full-scale floating platform would behave in the open ocean,” Lomonaco said.
Using robotics to simulate turbines
But something different was needed to determine the impact of wind. Because aerodynamic and hydrodynamic forces are measured using different scales that cannot be reconciled in physical models, wind forces had to be generated numerically. To accomplish that, the researchers employed a robotic arm to manipulate the mast of the scaled-down wind turbine that arises from the floating platform, explained Ted Brekken, a professor of electrical and computer engineering who focused on internal control systems for the study.
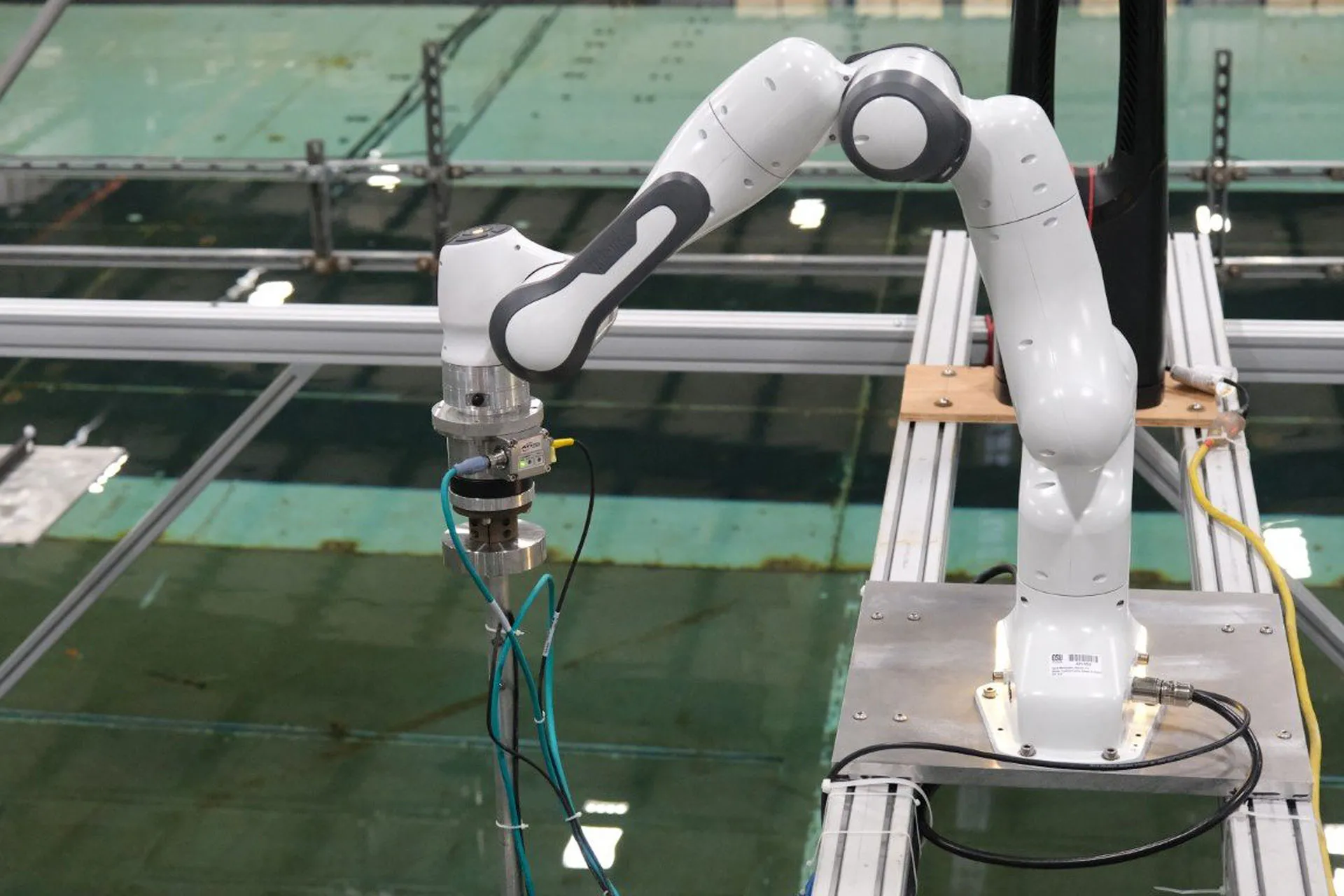
“The robotic arm, which is controlled by a computer running a wind turbine simulation, pushes and pulls the top of the tower and produces the same forces that a spinning turbine would,” Brekken said. “The rest of the system doesn’t ‘know’ that there isn’t a spinning turbine up there.”
“The hybrid capability of combining physical testing with numerical computation will greatly benefit research for floating offshore wind, because it can drive down the cost of testing the structures,” Robertson said.
During tests, however, the robotic arm was not able to adjust to the quickly changing motions of the system. Once funding for the next phase of the study becomes available, a more responsive robotic arm will be installed to improve the accuracy of the wave-wind-structure interactions.
“We were able to show that it’s possible to accurately replicate the motion of the platforms — aerodynamic forces included — in what we would call operational sea and wind states, with predictable winds and predictable waves,” Robertson said. “But what we really need to determine is what happens in heavy seas, strong winds, or catastrophic failures, like if a mooring line breaks or a turbine blade snaps. That’s the kind of information that will drive the actual structural design of full-size platforms.”
The West Coast’s wind energy future
The floating turbines envisioned for the West Coast will be enormous. The platforms are likely to span 100 meters or more and weigh thousands of tons. Their masts will reach 150 meters tall, and each turbine blade will be longer than a football field. The structures will reside 20 to 30 miles offshore in tracts leased by the federal government; even at those grand heights, they will not be overtly visible from the mainland.
Big and tall are desirable attributes for offshore wind turbines. The wind becomes more consistent as altitude increases, and the higher the blades reach, the more wind will hit them, which translates into lower costs per megawatt-hour, Robertson noted.
The DOE estimates that the cost of building and installing commercial-scale floating wind farms will be about 50% more than the cost of comparable fixed-bottom arrays, but that they will eventually become economically viable. And because larger turbines produce more energy than smaller ones, fewer units will be needed to generate more electricity, creating economies of scale and further suppressing costs. The energy industry expects that a single floating turbine off the West Coast will generate about 15 megawatts. Currently, the largest fixed-bottom offshore turbines produce about 6 MW, while typical onshore turbines produce 2-3 MW.
“Among the challenges for offshore wind is that the platforms are incredibly large, they’re incredibly expensive, and they require a significant amount of port infrastructure that just doesn’t exist yet on the West Coast,” Robertson said.
He calls the dilemma a technology option without an infrastructure solution, but there may be ways to change that.
Future opportunities and challenges
“Right now, floating offshore wind platforms are a version of oil and gas platforms with some version of a terrestrial wind turbine bolted on top,” Robertson said. “I think there are huge opportunities to look at new ways to design and build them as a single device from top to bottom that doesn’t require massive port infrastructure.”
Robertson and Lomonaco are quick to acknowledge that there is an ongoing discussion about the scale, timing, and applicability of offshore wind deployment in Oregon. Even with the most aggressive timelines, they anticipate that the first full-scale floating turbines won’t start operating off the West Coast for at least 10 years. In addition to the technological barriers that must be surmounted, other factors, such as social, environmental, and community concerns, also must be addressed.
“One of my goals as the director of PMEC is to broaden the engagement in our activities across the university so we aren’t perceived as just an engineering center, but as a place where we do a lot of work on ecological, social, and environmental fronts and on the business aspects of developing this technology,” Roberston said.
“Floating offshore wind power on the West Coast is going to happen with or without us at Oregon State,” Lomonaco said. “The difference is that by doing the work we’re doing here, we will be part of the process and contribute to solutions, taking into consideration technical, economic, and social aspects, so that wind power can become an even more important source of renewable energy.”